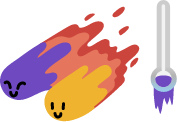
Pic 1. Bound electron pairs carrying super current called Cooper pairs.
Superconductivity is a property of specific materials, in which electrical resistance vanishes at low temperatures, making them not only superconducting, but also repellers for magnetic fields. At these very low temperatures the electrons inside the material pair up with one another becoming the charge carriers and replacing single electrons. To create powerful and energy efficient computing we need powerful ways to transfer electricity. Some of the most powerful magnets in NMR equipment for example are electromagnets made using superconductors.
The two distinguishing characteristics related to superconductivity were discovered at different times. Firstly it was discovered in 1911 that when dropping temperature below 4,15 K the resistance of mercury suddenly dropped to zero. The second feature, the perfect diamagnetism where magnetic flux is excluded in a cooled magnetic field (Meissner effect), was discovered later. The present theoretical understanding where bound electron pairs carrying super current are formed is based on discoveries made in the late 50’s.
In the heart of technological advancements of superconductivity is the research of different alloys and compounds of materials that can be used for resistance free energy flow. Superconductivity, roughly said, is a phenomenon that allows a macroscopic quantum coherent flow of electrons in a sufficiently cold piece of a fitting material, by establishing a specific order among them. It is an electronic state of lower, and more stable energy that possesses the interesting magnetic properties such as the ability to expel external magnetic fields (As portrayed with the experiment of levitating a magnet).
Fig 1. Use the thermometer to control the Cooper pair electrons. When a superconductor is placed in a weak external magnetic field and cooled below its transition temperature, the magnetic field is ejected.
A superconductor with little or no magnetic field within it is said to be in the Meissner state. The Meissner state however breaks down when the applied magnetic field is too large, even in a cooled environment. This is why superconductors can be divided into two classes according to how this breakdown occurs.
Type-I materials remain in the superconducting state only for relatively weak applied magnetic fields. Above a given threshold, the field abruptly penetrates into the material, shattering the superconducting state. In a type-II superconductor, the levitation of the magnet is further stabilized due to flux pinning within the superconductor; this tends to stop the superconductor from moving with respect to the magnetic field, even if the levitated system is inverted. A very strong magnetic field is required for example to levitate a train. The JR–Maglev trains have superconducting magnetic coils, but the JR–Maglev levitation is not due to the Meissner effect.
Electrons which usually repel each other prefer to condensate into the superconductive state in some materials because certain negatively charged electrons distort the neutral lattice of atoms and locally produce areas of positive charge instead. This induced effective charge attracts other electrons with matching momentum one to the other.
Such an indirect interaction between electrons therefore produces a new entity, the electron pair, that behaves like a new kind of particle called “Cooper pair”. Unlike individual electrons many Cooper pairs occupy the same energy state and have a zero spin, thus becoming a compound boson. Becoming a boson lets them undergo Bose condensation at low enough temperature. At this scale the microscopic quantum phenomena become apparent, such as the wave function interference.
Superconductors being able to conduct electricity without resistance, makes them valuable for long-distance electricity transmission and many other energy-saving applications. Conventional superconductors operate only at extremely low temperatures, but certain materials can superconduct at relatively high temperatures, for example graphene. A superconductor is generally considered high-temperature if it reaches a superconducting state above a temperature of 30 Kelvin (that’s -243,15 Celsius). For quantum computing to become fully realised the development is being done in finding a superconductor that can act in the same way as silicon does in today’s computing, as the chips, diodes and other electronic circuits.
More to read and links to text:
Elsevier Science & Technology 2007, Charles P., Jr. Poole, Richard J. Creswick, Horacio A. Farach and Ruslan Prozorov: Superconductivity
Phys.org Website 17 January 2019, Jade Boyd, Rice University: Physicists show quantum materials can be tuned for superconductivity https://phys.org/news/2019-01-physicists-quantum-materials-tuned-superconductivity.html
CERN website 2020: Superconductivity https://home.cern/science/engineering/superconductivity
Springer Graduate texts in Physics 2019, A. J. Berlinsky, A. B. Harris, Statistical Mechanics: An Introductory Graduate Course
Science Alert Webpage, 17 August 2019, David Bield: This Superconductor Could Be Key to a Whole Different Type of Quantum Computer. https://www.sciencealert.com/this-superconductor-material-could-be-the-silicon-of-quantum-computers
Cambridge University Press 2011, A. M. Zagoskin: Quantum Engineering: Theory and Design of Quantum Coherent Structures.
Text by Noora Heiskanen with special thanks to Silvia Cotroneo and Jani-Petri Martikainen